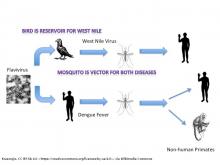
1. Premise
Preventing, controlling and mitigating the spread of disease is at the heart of public health work. Climate change may cause non communicable and communicable - or infectious - diseases. Examples of non-communicable diseases that may be induced by climate change are:
- Diseases that are caused by injuries;
- Cardio-vascular diseases (CVD);
- Neurological disorder.
Examples of infectious diseases are:
- COVID-19;
- flue;
- Lyme disease;
- malaria:
- Ebola.
1.1 Premise on noncommunicable diseases
Noncommunicable diseases (NCDs) kill 41 million people each year, equivalent to 74% of all deaths globally. Each year, 17 million people die from a NCD before age 70; 86% of these premature deaths occur in low- and middle-income countries. Of all NCD deaths, 77% are in low- and middle-income countries.
Cardiovascular diseases account for most NCD deaths, or 17.9 million people annually, followed by cancers (9.3 million), chronic respiratory diseases (4.1 million), and diabetes (2.0 million including kidney disease deaths caused by diabetes). These four groups of diseases account for over 80% of all premature NCD deaths. Climate change, tobacco use, physical inactivity, the harmful use of alcohol, unhealthy diets and air pollution all increase the risk of dying from an NCD.
1.2 Premise on communicable diseases
Outbreaks of infectious diseases can have an extraordinary impact on human health. The impact is strictly related to climatic conditions and therefore is affected by climate change.
In particular, vector-borne diseases are human illnesses caused by parasites, viruses and bacteria that are transmitted by vectors, that is, living organisms that move viruses, protozoans and bacteria from an infected body to another recipient of the infection. Every year there are more than 700,000 deaths from diseases such as malaria, dengue, schistosomiasis, human African trypanosomiasis, leishmaniasis, Chagas disease, yellow fever, Japanese encephalitis and onchocerciasis. Figure 1 reports a summary of reported case counts of notifiable vector-borne diseases in the United States.
An important sub-category of vector-borne diseases includes the water-born diseases (WBD), that are illnesses vehicled by microscopic organisms, like viruses and bacteria, that are ingested through contaminated water. People can get infected by WBD while bathing, washing, drinking contaminated water. Also, eating food that got in touch with contaminated water may expose to WBD.
Diarrhea and vomit are the most commonly reported symptoms of WBD. Other symptoms are problems at skin, ear, respiration, or eyes. Lack of clean water, sanitation and hygiene are major causes for the spread of WBD in a community. Therefore, reliable access to clean water and sanitation is the main method to prevent WBD.
Microorganisms causing WBD include protozoa and bacteria, many of which are parasites from the intestine.
Protozoa are a polyphyletic group of single-celled eukaryotes, either free-living or parasitic, that feed on organic matter such as other microorganisms or organic debris. Historically, protozoans were regarded as one-celled animals. See Figure 1.
Figure 1. Collage of protozoans, from images in Wikimedia commons. Clockwise from top left: Blepharisma japonicum, a ciliate; Giardia muris, a parasitic flagellate; Centropyxis aculeata, a testate (shelled) amoeba;
Peridinium willei, a dinoflagellate; Chaos carolinense, a naked amoebozoan; Desmerella moniliformis, a choanoflagellate. By Respectively: Frank Fox, Sergey Karpov, CDC/ Dr. Stan Erlandsen, Picturepest, Thierry Arnet, dr.Tsukii Yuuji - From Wikimedia Commons.
Bacteria are microbes with a cell structure simpler than that of many other organisms. Their control centre, containing the genetic information, is contained in a single loop of DNA. Some bacteria have an extra circle of genetic material called a plasmid rather than a nucleus. The plasmid often contains genes that give the bacterium some advantage over other bacteria. For example it may contain a gene that makes the bacterium resistant to a certain antibiotic.

Figure 2. Escherichia coli: Scanning electron micrograph of Escherichia coli, grown in culture and adhered to a cover slip. By Credit: Rocky Mountain Laboratories, NIAID, NIH - NIAID: https://commons.wikimedia.org/w/index.php?curid=104228
Other WBD are caused by viruses. WBD may also be caused by metazoan parasites. Examples include Nematoda, namely, "roundworms". Another example of WBD metazoan pathogens are Schistosomatidae, a family of blood flukes. They usually infect people that get their skin in touch with infected water. Blood flukes are pathogens that cause Schistosomiasis of various forms, affecting hundreds of millions of people worldwide.
The burden of vector-borne diseases is highest in tropical and subtropical areas. Their incidence is much higher among the poorest populations. Since 2014, major outbreaks of dengue, malaria, chikungunya, yellow fever and Zika have afflicted populations, claimed lives, and overloaded health systems in many countries. Other diseases such as Chikungunya, leishmaniasis and lymphatic filariasis cause chronic suffering, life-long morbidity, and disability.
This lecture focuses on climate related illnesses, which include several well-known and widely diffused pathologies, usually caused and aggravated by a complexity of factors, including climatic conditions.
We pay special attention to vectorn-borne and water-borne diseases, while other pathologies are described more synthetically. In particular, the impact of climate change on non-communicable diseases is more easily decipherable with respect to infectious diseases, which depend on more complex and numerous factors. We make special reference to "Climate change for health professionals - A pocket book", by Pan American Health Organization and World Health Organization.
2. Vector-borne diseases
Vector-borne diseases are influenced significantly by climate factors, including high and low temperature extremes and precipitation patterns. Thus, changes in climate and weather patterns modify the distribution of diseases and their outbreaks through changes in biological systems and processes, such as vector population size and density, vector survival rates, the relative abundance of disease-carrying animal (zoonotic) reservoir hosts, and pathogen reproduction rates. The impact of climate change is not necessarily negative: in some cases one may find benefits from changes in climate. However, climate change always results in a perturbation of the equilibrium that communities typical find - through adaptation - with the health risks. Therefore, in most of the cases further adaptation is needed after climate change.
The interaction of climate change with vector borne diseases is only partially known and therefore any modelling and interpretation exercise is subjected to large uncertainty. This is due to the casual nature of several of these processes, and also the presence of several confounding factors and additional drivers besides climate.
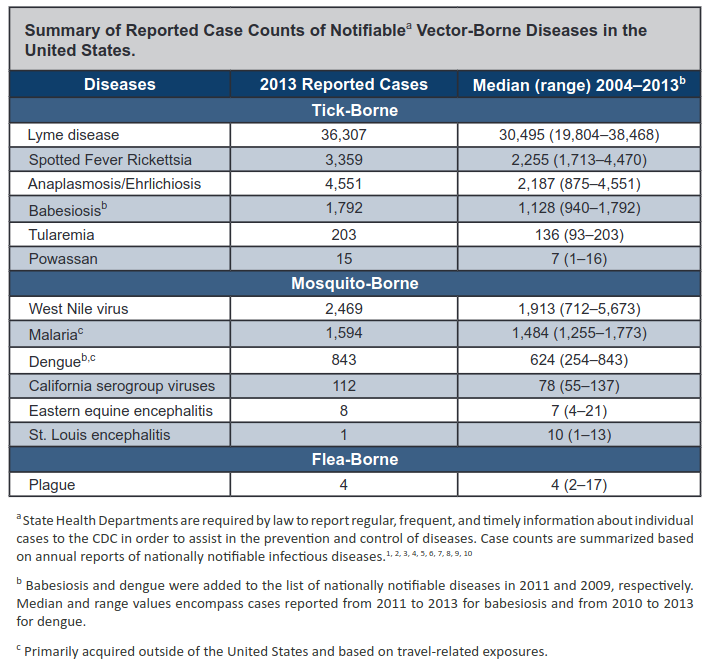
Figure 3. Summary of reported case counts of notifiable vector-borne diseases in the United States. Source: USGCRP (2016), chapter 5. References for the diseases in the table that were not earlier mentioned: Spotted Fever Rickettsia, Anaplasmosis/Ehrlichiosis, Babesiosis, Tularemia, Powassan, California serogroup viruses, Eastern equine encephalitis, St. Luois Enecephalitis.
Climate change is expected - with high confidence - to cause an increase of global temperature and changes in seasonality. After global warming, Spring is anticipated and the Fall season is delayed. Therefore, the life cycle of vectors is changed as well, with a likely increase of their period of activity and a corresponding increase of their number and diffusion.
2.1. Lyme disease
A relevant example is given by the increasing spread of ticks which may convey the Lyme disease, the most common vector-borne disease in the United States. The example is illustrated here in detail, by also discussing models for assessing the impact of climate change. Similar approaches can be applied to the case of other infectious diseases.
Lyme disease is caused by the bacterium Borrelia burgdorferi and rarely, Borrelia mayonii. It is transmitted to humans through the bite of infected blacklegged ticks. Typical symptoms include fever, headache, fatigue, and a characteristic skin rash called erythema migrans. If left untreated, infection can spread to joints, the heart, and the nervous system. For more details see, for instance, here.
The seasonal occurrence of Lyme disease cases is related, partially, to the timing of a blood meal (host-seeking activity) of ticks and the three-stage life cycle (larvae, nymph, and adult) of ticks. Increasing temperatures and the accompanying changes in seasonal patterns are expected to result in earlier seasonal tick activity and an expansion in tick habitat range, increasing the risk of human exposure to ticks (Figure 4).
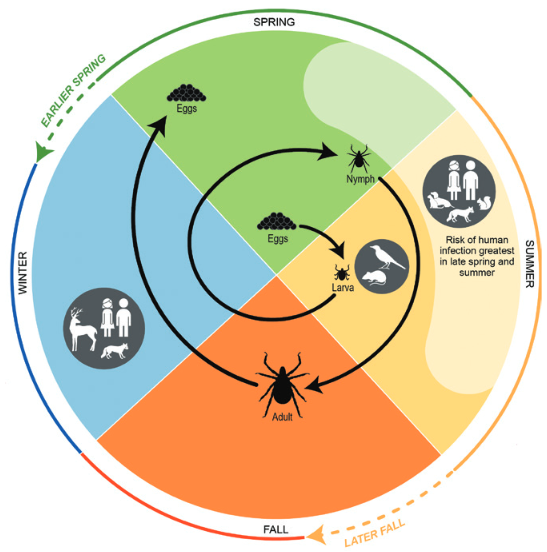
Figure 4. Life cycle of blacklegged ticks, including the phases in which humans can be exposed to Lyme disease, and some of the changes in seasonality expected with climate change. Source: USGCRP (2016), chapter 5, adapted from CDC (2015).
Ticks may get infected by feeding on rodents, other mammals and birds during Spring and Summer. The infection can then be passed to humans through the bite of the ticks. The longer the permanence of the tick on humans, the higher the risk of infection.
Aside from short periods that they spend on the host, the ticks pass much of their time in the natural environment, in grass fields or forests. The weather condition have a strong influence on the probability to survive and find hosts. In general they suffer the too hot and too cold weather and generally benefit from humidity. The geographic distribution of ticks and infected ticks are essential parameters conditioning the risk for human health. Monitoring and education play an essential role to mitigate the risk.
After climate change, warmer winter and spring temperatures are projected to lead to earlier annual onset of Lyme disease cases in the eastern United States and in an earlier onset of host-seeking behavior.
An analysis of climate change impact on the annual onset of Lyme disease in the United States has been carried out by Monaghan et al. (2015). To estimate the Lyme onset week (counted by assuming that week 1 is located at the beginning of the calendar year), namely, the week with the maximum increasing gradient in the number of Lyme disease cases, they use the following relationship:
LOW = 17.563 - 0.014 GDDW20 + 0.945 SDM5 + 0.09 PRCPAW8 + 0.093 DIST
where LOW is Lyme onset week, GDDW20 is the cumulative growing degree days from week 1 (a tick growing degree day is identified by a mean value of daily temperature above 10 °C), SDM5 is the mean saturation deficit in the atmosphere in mmHg in the 5 weeks before the onset week, PRCPAW8 is cumulative rainfall in mm from week 8 and DIST is distance in decimal degrees to the Atlantic Ocean coastline from the weighted mean center of each State’s total Lyme disease cases.
The model performances were quite good, with an adjusted R2 of 0.78. As the above equation shows, the critical week is anticipated by large values of GDDW20, while it is delayed by higher saturation deficit, cumulative rainfall (higher rainfall usually corresponds to lower temperatures) and increasing distance from the coast.
Clearly, temperature is a significant explanatory variable of the above model. The authors went on to assess the evolution of LOW according to climatic projections for different emission scenarios. The results are summarised by Figure 5.

Figure 5. Impact of climate change on the onset of Lyme disease in the United States. From Monaghan et al. (2015). For additional details see the original caption above.
The authors conclude that:
A climate-based empirical model was driven with an ensemble of downscaled CMIP5 climate model simulations to project the impacts of climate change on the annual onset week of Lyme disease nationally and for 12 States. The average LOW is projected to become 0.4–0.5 weeks earlier nationally for 2025–2040 (p < 0.05), and 0.7–1.9 weeks earlier for 2065–2080 (p < 0.01). The smallest changes are projected for the lowest greenhouse gas emissions scenario, RCP2.6, while the largest changes would occur for the highest greenhouse gas emissions scenario (RCP8.5). Warming temperatures during the winter and spring months increase GDDW20 and cause earlier LOW in the future. Projected increases in SDM5 and PRCPAW8 both partially offset the effects of warming on LOW.Regionally, the mid-Atlantic States are projected to have larger changes in LOW compared to the northern States, a result the historical record supports. The results of the present study suggest that 21st century climate change, particularly increasing temperatures, will likely make environmental conditions suitable for earlier onset of Lyme disease cases in the United States.
2.3. West Nile Virus
West Nile virus (WNV) is the leading cause of mosquito-borne disease in the continental United States. It is typically spread to people by the bite of an infected mosquito. Cases of WNV occur during mosquito season, which starts in the summer and continues through fall. There are no vaccines to prevent or medications to treat WNV in people. Fortunately, most people infected with WNV do not feel sick. About 1 in 5 people who are infected develop a fever and other symptoms. About 1 out of 150 infected people develop a serious, sometimes fatal, illness.
From 1999 to 2013, a total of 39,557 cases of WNV disease were reported in the United States. Annual variation is substantial, both in terms of case counts and the geographic distribution of cases of human infection (USGCRP, 2016).
West Nile Virus is transmitted through cycles of infections between birds and mosquitos. Human infection may occur with the bite of a mosquito that has previously bitten an infected bird. Humans do not pass infections to biting mosquito because humans do not have sufficient concentration of the virus in their bloodstreams. The chain of the infection and climate impact are shown in Figure 6.
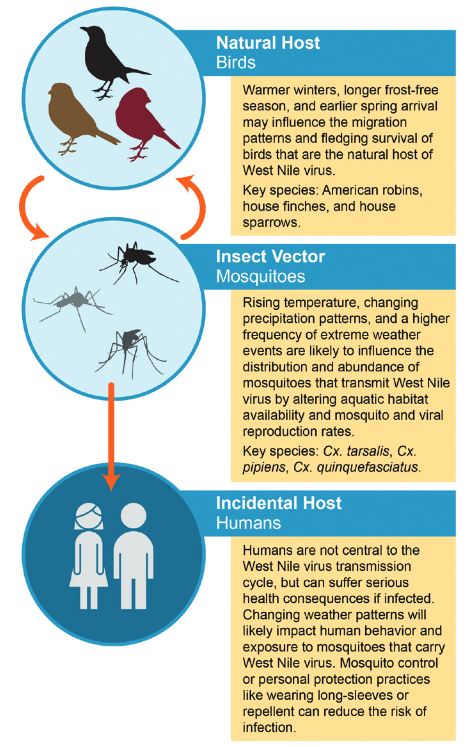
Figure 6. Climate impacts on West Nile virus transmission. From USGCRP (2016).
Climate impacts are determined by the effect of temperature and humidity - thus precipitation - on the life cycle of birds and mosquitos. Again, raising temperature and raising humidity are expected to increase the onset of WNV.
2.4. Vibrio and other water-born infections
Vibrio (genus Vibrio) is a group of comma-shaped bacteria in the family Vibrionaceae. Vibrios are aquatic microorganisms, some species of which cause serious diseases in humans and other animals. Vibrios are microbiologically characterized as gram-negative, highly motile, facultative anaerobes (not requiring oxygen), with one to three whiplike flagella at one end. Their cells are curved rods 0.5 μm (micrometre; 1 μm = 10-6 metre) across and 1.5 to 3.0 μm long, single or strung together in S-shapes or spirals.
Three species of vibrio are of significance to humans: V. cholerae is the cause of cholera, and V. parahaemolyticus and V. vulnificus both act as agents of acute enteritis, or bacterial diarrhea. V. anguillarum is found in diseased eels and other fishes.
Being Vibrio aquatic organisms, they are clearly influenced by climate change and in particular by the water cycle: precipitation, humidity, evaporation, infiltration and river flow. The presence of Vibrio is also related to water quality and therefore to the state of urban quality, sewer system, water treatment and so forth.
Health outcomes are determined by different exposure pathways and multiple other social and behavioral factors, some of which are also affected by climate. Research is focusing on understanding how climate drivers affect physical and ecological processes that act as key exposure pathways for pathogens and toxins, as shown by the arrow moving from the top to the middle box in Figure 7.

Figure 7. Conceptual diagram for an example of infection by Vibrio species. From USGCRP, chapter 6.
Table 1 shows various health outcomes that can result from exposure to agents of water-related illness (including vibrio) as well as key climate-related changes affecting their occurrence.
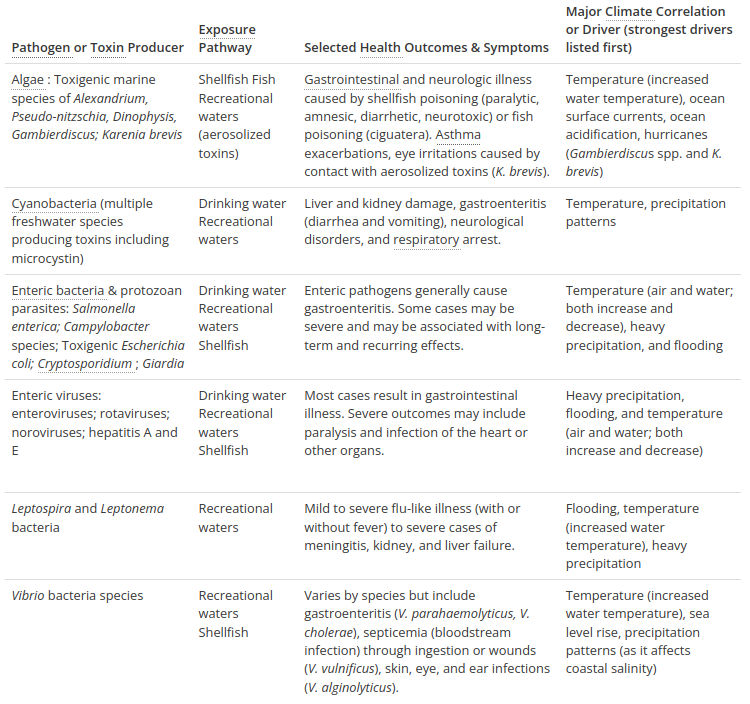
Table 1. Health outcomes that can result from exposure to agents of water-related illness (including vibrio) as well as key climate-related changes affecting their occurrence. From USGCRP, chapter 6.
The primary sources of water contamination are human and animal waste and agricultural activities, including the use of fertilizers. Runoff and flooding may increase risks of contamination, as the sewer systems may overflow and the water treatment is usually not sized to treat huge water flows. In fact, contamination occurs when agents of water-related illness and
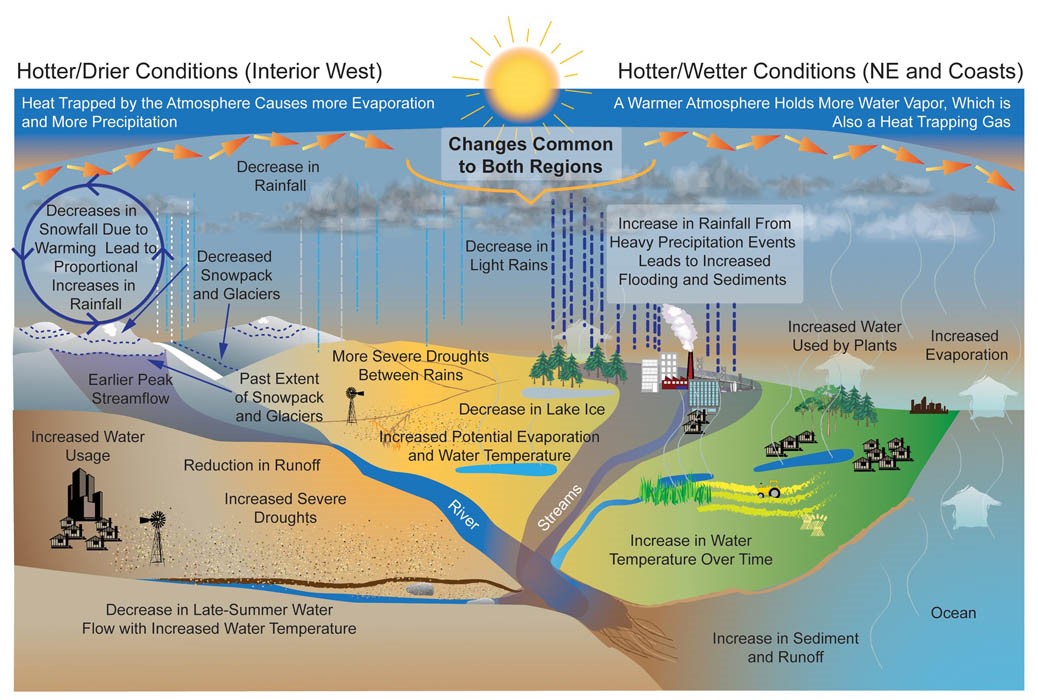
Figure 8. Projected changes in the water cycle. Source: USGCRP 2009 and Environmental Protection Agency.
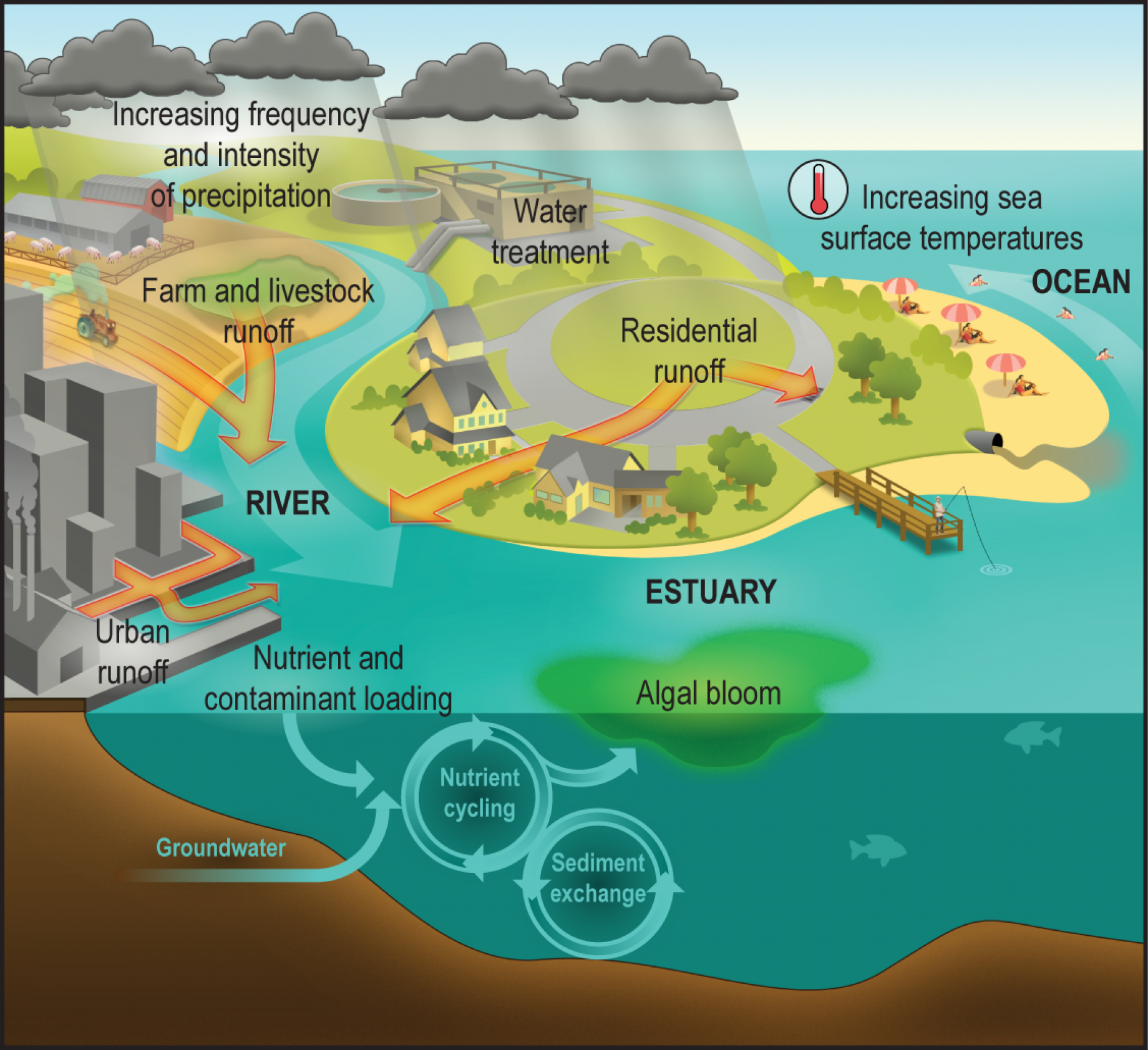
Figure 9. Climate change, water borne illnesses and human exposure. Source: National Institute of Environmental Health Sciences, https://www.niehs.nih.gov/
To clarify how contamination may occur, it may be useful to recall how sewer systems in urban areas usually work. Most of the existing sewer systems are "combined" systems: in other words, they collect in the same pipe the waste water and the rainfall water drained from urban areas. Only recently separate sewer systems were increasingly adopted.
During dry periods, the flow in the combined sewer system is dominated by wastewater and is generally low. It conveyed entirely at the treatment plant. During storm, flow is dominated by urban drainage and increases a lot, thus exceeding the capacity of the treatment plant. Therefore, combined systems are designed in order to release the excess water directly into surface water. Even if the wastewater is diluted, still the water that is directly released in the river network is infected and may cause an onset of the infection.
A striking example of contamination from human wastes is given by the 1854 Broad Street cholera outbreak in London, during the European cholera pandemic. At that time, the causes of cholera were unknown. The cholera-causing bacterium Vibrio cholerae was isolated in 1854, but the finding did not become well known and accepted until decades later. During the 1854 outbreak a detailed mapping of the cholera cases was carried out by John Snow. He discovered that most of the cases were concentrated around the Broad Street pump, a public water supply fed by groundwater that got contaminated by human waste.
To better assess the interaction between water and humans, combined models have been recently developed to simulate the dynamics of water flow in the river network, depending on precipitation and other hydrological variables, along with water quality, depending on the human pressure and sewer flow and overflow. These models allow to infer the impact of climate change on infectious diseases.
Similar models can be used to predict the impact of agricultural contamination. Management practices and technologies, such as better timing of manure application and improved animal feeding, help mitigate or eliminate the risk of manure contamination and the spread of contaminants to public water supplies, shellfish harvesting waters and reduce nutrients that stimulate harmful algal blooms. Drinking water treatment and monitoring practices also help reduce or eliminate exposure to water-borne disease from agricultural environments.
The above discussion has highlighted the prominent role of water and water flow in spreading Vibrio infections including cholera. In fact, several researchers were recently carried out to investigate the key role of the river network and river flow for the propagation of cholera (Bertuzzo et al., 2009; Rinaldo et al., 2012). These models take into account the local propagation of susceptible individuals and infectious agents in a network of nodes linked by networks (like river network and road network) having different topologies. In fact, the river network regulates both the displacement of humans and the propagation of agents. Thus, the vehicle of infection (Vibrio cholerae) is transported through the network links which modelled as hydrological connections among susceptible communities. These models have been proved to be capable of predicting the evolution of infections. They are instrumental for the risk control and mitigation.
Public health safeguards can mitigate the risk carried by waterborne pathogens and toxins and limit outbreaks of water-related illnesses. It is essential to monitor water quality continuously and in particular after extreme weather events. Policies setting water quality standards for drinking water and issuing advisories for consuming fish and shellfish are usually adopted in many countries.
Ecosystems play an essential role as they contribute to the degradation of contaminants. Restoring and protecting wetlands can also help reduce greenhouse gases through carbon sequestration. Promoting safe water quality can be accomplished by capturing and treating contaminated runoff, temporary storage of overflows from sewer systems, and natural filtration of contaminants.
2.5. Covid-19
We don’t have solid evidence that climate change influenced the onset and spread of COVID-19. However, the question was raised on whether the recent changes in climate, in particular global warming, had a significant role to trigger the "species jump" that might have originated the pandemic. Indeed, we know that climate variability and change alters how we relate to other species on Earth and that matters to our health and our risk for infections.
As the planet is warming up, living species are forced to move (temporarily or permanently) to seek more favorable living conditions. In particular, animals may be forced to move from their habitat therefore getting more connected to humans, thus favoring the onset of zoonotic diseases (Bale, 2020; Xiao et al., 2020).
Futhermore, Covid-19, like flue, is sensitive to weather conditions. The trajectories of contagion often exhibit strong seasonal patterns with fewer cases during summer and more during winter. A range of studies provide support to the empirical evidence for the negative relationship between temperature and contagion. However, other studies come to contrasting conclusions, showing that particular local conditions may lead to different outcomes.
Several other consequences of climate change also increase the risk of pandemics. Deforestation, which occurs mostly for agricultural purposes, is the largest cause of habitat loss worldwide. Loss of habitat forces animals to migrate and potentially contact other animals or people and share germs. Large livestock farms can also serve as a source for spillover of infections from animals to people.
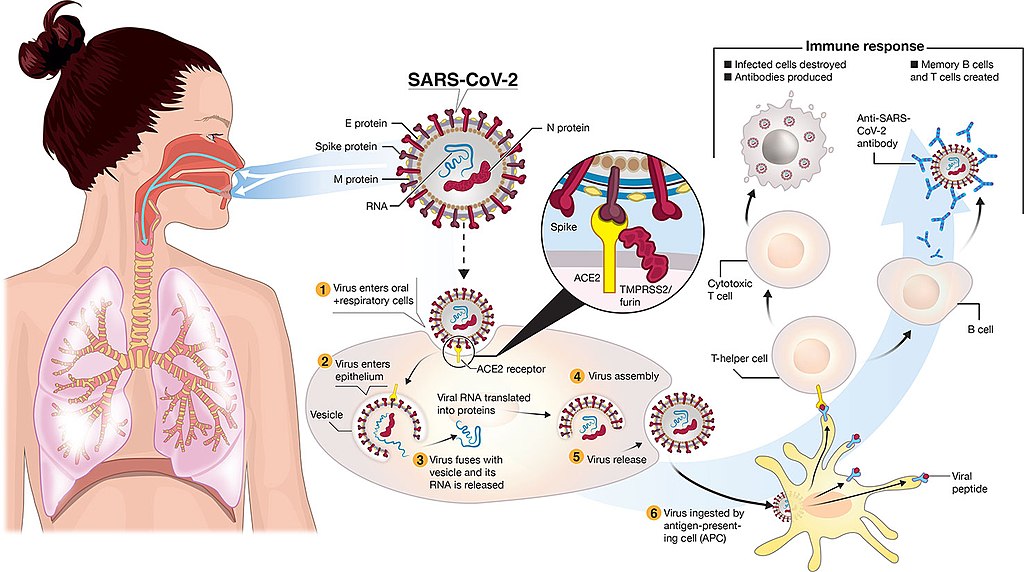
Figure 10. Transmission and life-cycle of SARS-CoV-2 causing COVID-19. Source: Colin D. Funk, Craig Laferrière, and Ali Ardakani. Graphic by Ian Dennis - http://www.iandennisgraphics.com, CC BY 4.0, via Wikimedia Commons
3. Cardiovascular diseases
Cardiovascular diseases are impacted by several climatic variables including temperature, humidity, air pollution. Moreover, illnesses and symptoms may be aggravated by the occurrence of natural disasters like hurricanes, floods, droughts. These events may cause stressful situations impacting the blood pressure, the hearth beat etc. The pathophysiology of cardiovascular diseases is schematically presented in Figure 11 (from "Climate change for health professionals - A pocket book", by Pan American Health Organization and World Health Organization).
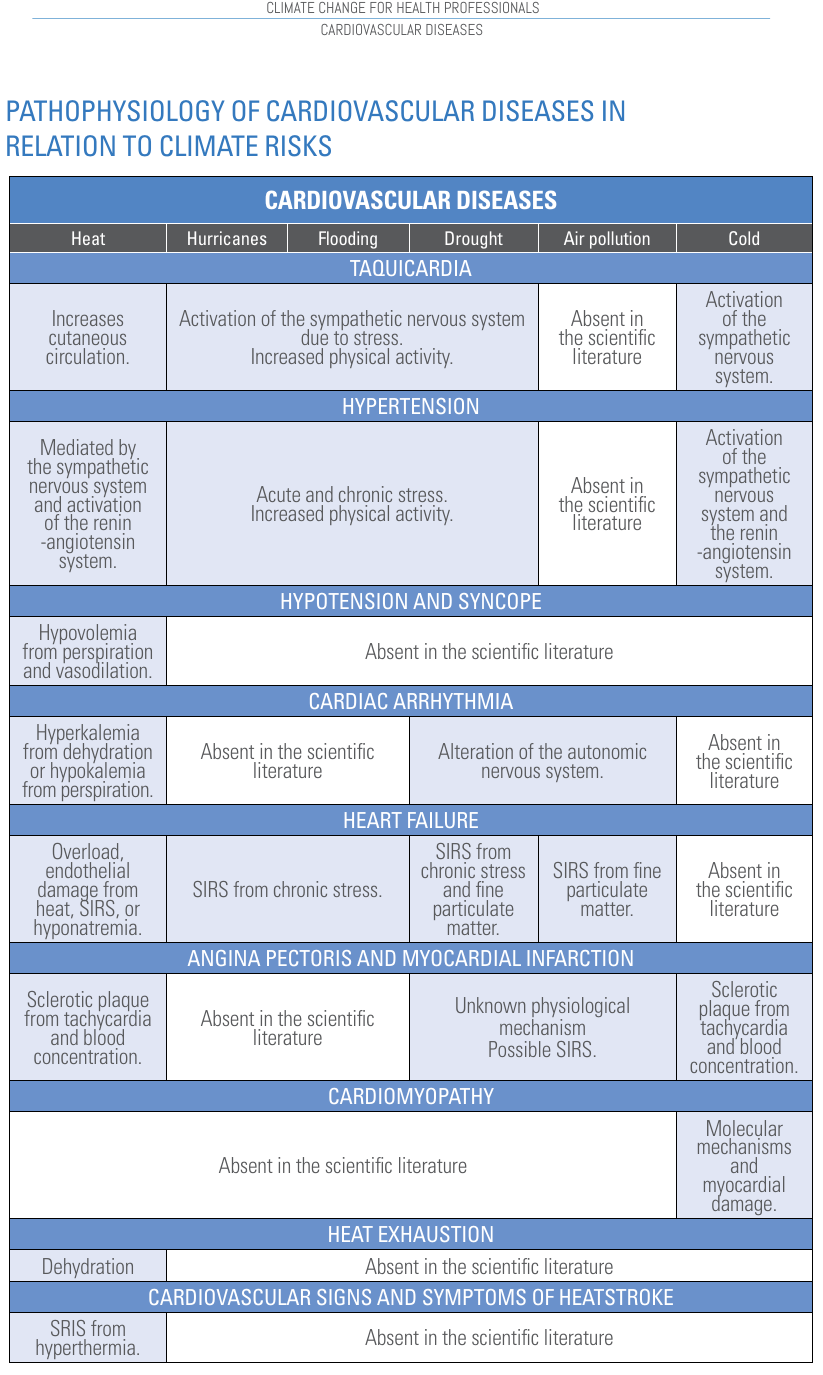
Figure 11. Pathophysiology of cardiovascular diseases. SIRS is Systemic Inflammatory Response Syndome. Source: "Climate change for health professionals - A pocket book", by Pan American Health Organization and World Health Organization.
4. Respiratory diseases
Climatic variables and extreme events caused by climate may have a significant impact on human respiratory health. Poor air quality and increased allergens can worsen existing disease and cause new pathologies. The biological mechanisms triggering respiratory diseases when one is exposed to climate change and climate risks are still unclear. Our current knowledge is schematically presented in Figure 12.

Figure 12. Pathophysiology of respiratory diseases. Source: "Climate change for health professionals - A pocket book", by Pan American Health Organization and World Health Organization.
5. Neurological disorder
Climate change associated to extreme environmental conditions may induce neurological impairment and increase stroke incidence and mortality. Increasing temperature does not participate in the etiology of neurodegenerative disorders, but it may exacerbate symptoms of dementia, Alzheimer's disease (AD) and Parkinson's Disease (PD). Indeed, some patients might find their symptoms worsen: multiple sclerosis may be affected by temperature, as some types of epilepsy. Neurological diseases linked to vectors, such as cerebral malaria, Zika virus, Japanese encephalitis, and neurocysticercosis, are likely to increased their incidence under global warming. In the most recent Global Burden of Diseases and Risk Factors study on stroke, non-optimal temperatures, whether hot or cold, were associated with increased risk, and air pollution was one of the top five risk factors for stroke. In the 2020 Lancet commission on dementia, air pollution was one of three newly identified modifiable risk factors.
Our current knowledge on climate change and neurological disorder is schematically presented in Figure 13.

Figure 13. Pathophysiology of neurological disorders. Source: "Climate change for health professionals - A pocket book", by Pan American Health Organization and World Health Organization.
6. Mental health disorder
Exacerbation of weather extremes may lead to the occurrence of extreme events like floods, drought, hurricanes and so forth. These events generate stressful situations. As a result, individuals may experience higher levels of psychological distress and a minority may develop more serious mental health problems, such as post-traumatic stress disorder (PTSD), depression, or substance use disorders.
The Pan American Health Organization and World Health Organization present the schematic copied in Figure 14 to summarise the impact of climate change on mental health disorder. Our knowledge on these impacts is still limited and evolving.

Figure 14. Pathophysiology of mental health disorders. Source: "Climate change for health professionals - A pocket book", by Pan American Health Organization and World Health Organization.
7. Skin diseases
Higher temperatures and humidity can lead to more sweating, triggering flare-ups for individuals with eczema and psoriasis. It can also lead to other skin conditions such as rashes, athlete's foot, and hives. In regard to this, The Pan American Health Organization and World Health Organization present the schematic copied in Figure 15.

Figure 15. Pathophysiology of skin diseases. Source: "Climate change for health professionals - A pocket book", by Pan American Health Organization and World Health Organization.
8. Other diseases
The Pan American Health Organization and World Health Organization, in the pocket book "Climate change for health professionals" also mentions the possible impact of climate change on kidney, eye, gastrointestinal, maternal and pediatric disorders.
9. Advice for patients and communities
The above discussion has highlighter several possible connections between climate change and many types of illnesses. Some of these connections are still poorly known. These related to temperature and air pollution are relatively well understood, while the dependence of illnesses from extreme events is still the subject of ongoing research. The impact of climate change on extreme events themselves is still poorly known and varying depending on location, season and socio-economical context.
Then, the most important advice to give the patients and communities is to get prepared and educated on what is known about climate change in their region, what the impact on extreme events is expected to be and to what extent the climate risks are relevant and increasing. Information avoids to undergo anxiety and therefore stressful situation.
Furthermore, a useful advice is to reduce the exposure of individuals and communities to the negative effects of climate. This is appropriate for all people, but especially those who are more exposed or particularly sensitive to climate risks. Although it is impossible to control the imminent occurrence of weather events that threaten human health, one can try to avoid them. For example, being informed of the weather forecast helps deciding to either evacuate the danger zone or make the appropriate preparations.
Specific advice for different types of risk are given by the pocket book "Climate change for health professionals" (see reference list).
References
Bale, R. (2020). Trafficked pangolins can carry coronaviruses closely related to pandemic strain. Scientific American, 322.
Bertuzzo, E., Casagrandi, R., Gatto, M., Rodriguez-Iturbe, I., & Rinaldo, A. (2010). On spatially explicit models of cholera epidemics. Journal of the Royal Society Interface, 7(43), 321-333.
CDC, 2015: Ticks: Life Cycle of Hard Ticks that Spread Disease. Centers for Disease Control and Prevention, Atlanta, GA. http://www.cdc.gov/ticks/life_cycle_and_hosts.html
Monaghan, A.J., S.M. Moore, K.M. Sampson, C.B. Beard, and R.J. Eisen (2015). Climate change influences on the annual onset of Lyme disease in the United States. Ticks and Tick Borne Diseases, 6, 615-622. http://dx.doi.org/10.1016/j.ttbdis.2015.05.005
Pan American Health Organization and World Health Organization (2020). "Climate change for health professionals - A pocket book".
Rinaldo, A., Bertuzzo, E., Mari, L., Righetto, L., Blokesch, M., Gatto, M., ... & Rodriguez-Iturbe, I. (2012). Reassessment of the 2010–2011 Haiti cholera outbreak and rainfall-driven multiseason projections. Proceedings of the National Academy of Sciences, 109(17), 6602-6607.
USGCRP, 2016: The Impacts of Climate Change on Human Health in the United States: A Scientific
Assessment. Crimmins, A., J. Balbus, J.L. Gamble, C.B. Beard, J.E. Bell, D. Dodgen, R.J. Eisen, N. Fann, M.D. Hawkins, S.C. Herring, L. Jantarasami, D.M. Mills, S. Saha, M.C. Sarofim, J. Trtanj, and L. Ziska, Eds. U.S. Global Change Research Program, Washington, DC, 312 pp. http://dx.doi.org/10.7930/J0R49NQX
Xiao, K., Zhai, J., Feng, Y., Zhou, N., Zhang, X. U., Zou, J.-J., Li, N. A., Guo, Y., Li, X., Shen, X., Zhang, Z., Shu, F., Huang, W., Li, Y. U., Zhang, Z., Chen, R.-A., Wu, Y.-J., Peng, S.-M., Huang, M., … Shen, Y. (2020). Isolation of SARS-CoV-2-related coronavirus from Malayan pangolins. Nature, 583(7815), 286–289. https://doi.org/10.1038/s41586-020-2313-x
Download the Powerpoint presentation of this lecture.
Last modified on November 15, 2023
- 82 viste